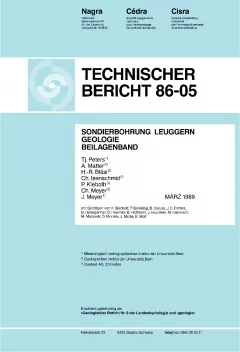
Technischer Bericht NTB 86-05
Sondierbohrung Leuggern:Geologie
The Leuggern borehole was drilled in the Plateau Jura of Canton Aargau. The drill-site lies on land administered by the borough of Leuggern, and is located some 13 km to the north of Brugg (G.R. 657.664, 271.208; altitude 358.8 m above sea level). Drilling work lasted form July 9th, 1984, until February 10th, 1985. Isolated short sections were drilling using a centre-bit, but otherwise a roller-bit was used exclusively and the sedimentary cover and crystalline basement were cored virtually continuously down to the maximum depth of 1688.90 m.
The 222.26 m – thick sedimentary succession is subdivided into Quaternary (48.50 m), Upper, Middle, and Lower Muschelkalk (39.37 m, 71.21 m, and 48.10 m thick respectively) and Buntsandstein (15.58 m).
The Upper Muschelkalk includes approximately 10 m of Trigonodus Dolomite in fully dolomitised Plattenkalk facies. Drusy cavities are filled with honey-yellow calcite; solution vugs are also present and show calcite and gypsum fillings. The Hauptmuschelkalk is 29.65 m thick and contains porous beds packed with crinoid ossicles. The horizons display secondary mouldic porosity after bivalves, much of which is infilled by cm-sized zoned calcite or dolomite crystals. The Middle Muschelkalk includes the Dolomit der Anhydritgruppe (12.52 m), which shows several phases both of solution and of precipitation of authigenic minerals such as quartz, talc, gypsum, calcite, fluorite and pyrite. Also included in the Middle Muschelkalk are the Upper Sulfatschichten (46.44 m), the Salzlager (salt horizon; 7.88 m) and the Lower Sulfatschichten (4.37 m). The facies and lithostratigraphy of the Upper Sulfatschichten are similar to and correlatable with those of the other Nagra boreholes; as at Böttstein the upper part of the Upper Sulfatschichten is gypsified.
The Lower Muschelkalk is developed in its typical uniform mud-marl facies. The Buntsandstein includes: sandy, dolomitic marly mudstones (the "Röt"); small-scale cycles of muddy, fine to coarse, pale green to white sandstones, together with mottled muddy sandstones (these form the Upper Buntsandstein); violet nodular sandstones with jasper and sandy caliche nodules ("Cargneule horizon”); and, at the base, cross-bedded coarse white sandstones (the "Diagonal bedded sandstone"). The Buntsandstein is also characterised by a complex diagenetic history; pedogenic processes were dominantly responsible for the formation of gravelly and calcareous horizons which interrupt the primary sand succession.
The basement (22.76 m drilled) lies beneath the Buntsandstein, which rests in sharply discordant contact above the basement. Slight weathering of the palaeosurface is evident in the topmost 5 m of the basement. Drilling revealed the presence down to 1387.3 m of a heterogeneous succession of gneissous metasediments dominated by metapelites and metagreywackes. This succession is interrupted by calcsilicate layers and banded sequences containing amphibolites and aplitic gneisses of volcano-sedimentary origin. The gneisses are highly metamorphosed and show evidence of the early stages of migmatisation. The rocks probably record Precambrian sedimentation. The succession is comparable with gneisses from the Kaisten borehole and especially with Vorwaldscholle (southernmost Black forest) gneisses. Granite was drilled from a depth of 1387.3 m down to the base of the borehole at 1688.90 m. The granite-gneiss contact is sharp and indications of contact metamorphism are absent. The granite is chiefly of porphyritic, biotite-granite but includes a section of two-mica granite in the lower part of the borehole. The granite belongs to the same pluton as the Böttstein Granite. Vein rocks (e.g. aplite, pegmatite, lamprophyre, quartz and tourmalinequartz veins) appear mostly in the gneiss series. The lamprophyre vein swarms seem to predate the granite.
The crystalline basement at Leuggern was overprinted by several post-metamorphic, post-granitic, tectonic-hydrothermal events. Post-metamorphic ductile deformation is evident from shear zones, which are absent in, and thus older than, the granite. Intrusion of the granite in the Carboniferous was associated with strong cataclastic deformation and high-temperature hydrothermal modification of the gneisses. Fluid inclusion studies show that the resultant high geothermal gradients above the still-hot granite led to convective circulation of relatively low-salinity NaCI-bearing aqueous fluids. The consequent fluid overpressures resulted in microfracturing and boiling phenomena now recorded by the fluid inclusions. The processes affecting the granite were thus associated with the hydrothermal action rather than with the deformation zones. Later, low temperature conditions in the early Permian were accompanied by renewed phase of intense fracturing of the crystalline basement and penetration from the palaeosurface of highly saline, CaCl2-charged oxidising aqueous fluids. These fluids accomplished alteration of the rocks to clay. In the gneisses, this alteration occurred only locally, and along deformation zones; in contrast, alteration was almost penetrative in the granites. In addition, alteration caused the Rb-Sr isotope system of the entire granite to re-equilibrate; late illites give K-Ar ages (279 ± 5 Ma) similar to these provided by the Rb-Sr whole rock isochrons (4 samples). The modification to clay also produced marked changes in the physicochemical rock characteristics.
Later events are recorded by solution cavities and mineral fracture fillings which grew freely into open fractures. In most cases, the mineralogy of the fracture fillings does not record geochemical equilibrium with modern formation waters. In accordance with this, it seems that the U- and Th-decay series have experienced no geochemical rock-water exchange since about 1.5 Ma BP. The exchangeable cations in the clay mineral interlayers likewise do not reflect modern formation water chemistry. In contrast with the Böttstein borehole, no depth zonation was recognised at Leuggern either in the clay minerals or in the geochemistry. This suggests the operation of more-or-Iess discrete alteration zones extending to a depth of 1500 m along faults, veins, or even along fracture systems.
Despite problems with orientation of the core sketches, structural analyses yielded interesting results. The gneiss foliation dips fairly constantly from 20 – 45° between SW and NW. Throughout the crystalline basement, the aplite veins dip 50 – 55° to the E or ENE and are thus oriented approximately perpendicular to the gneiss foliation. The quartz- and quartz-tourmaline veins in the granite form steeply inclined to subvertical NW-SE to WNW-ESE vertical striking structures. In the gneiss, deformation zones seem mostly perpendicular to the gneiss foliation and thus run subparallel to the aplite veins, while in the granite the deformation zones are remarkably parallel to the quartz- and quartz-tourmaline veins. 97.5 % of the 18'165 fractures encountered were sealed; 2.5 % were open fractures. The average fracture frequency is 13 per metre in the gneiss series and 9 per metre in the granite. Most of the fracturing was produced as a result of the Permian tectonic-hydrothermal overprinting. The fracture fillings permit identification of different fracture generations. There are significant differences between the fracture fillings in the gneiss series and those in the granite, although claymineral- and calcite-bearing fillings are by far the most common in both. No clear relationship between depth and fracture-fill mineralogy was discernible. The spatial orientation of the fractures is different in the gneiss series from in the granite; whereas in the gneiss series the fractures are primarily subparallel to the gneiss foliation or else occasionally run subparallel to the aplite veins, the fractures in the granite are oriented along the older quartz- and quartz-tourmaline veins. It is clear that pre-existing structures also characteristically exerted strong control on fracture development.
The following petrophysical parameters were determined for the various basement lithologies: rock and grain density; total and effective porosity; internal and external specific surface and thermal conductivity. The effect of the hydrothermal alteration on these parameters is generally considerable.
The localisation of water inflow was accomplished by means of "fluid logging" and core inspection. A total of 35 inflow points were registered, of which 29 were clearly indentifiable on the core itself (including all the major ones). Four different types of flow system could be characterised:
- 35 – 40 % of the inflow points are provided by open cavities, channels and fractures, either within, or in the close vicinity of, cataclastic deformation zones. This type of flow system is of lesser importance in the granite;
- 40 – 45 % of the inflow points occur at open fractures in the gneisses not directly related to cataclastic deformation zones;
- Open fractures within acid vein rocks provide 5 – 15 % of the inflow points. This system was only observed in the granites;
- The fourth, previously unobserved, type of flow system is provided by subvertical quartz-barite veins with open fractures and vugs. This flow system occurs in the granite, being responsible for the significant water inflow between depths of 1648 m and 1689 m, and is not accompanied by cataclastic zones (ca. 5 %).