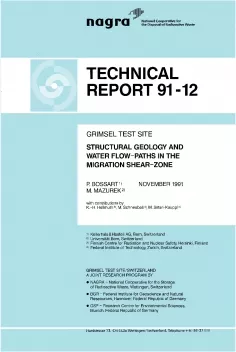
Technical Report NTB 91-12
Grimsel Test SiteStructural geology and water flow paths in the migration shear zone
Introduction: The shear-zone in the ventilation tunnel at VT 420 at the Grimsel Test Site (GTS) is a direct continuation of the migration shear-zone AU 96 that is being used for hydraulic and tracer migration experiments. A thick drill-core (diameter 20 cm) was taken from the VT 420 location such that the fragile structures in the central fault breccia remained largely intact. After several hardening steps, thin-sections were produced for all three main deformation planes of the deformation ellipsoid (i.e. parallel/perpendicular to the cleavage and stretching lineation). Vacuum impregnation with fluorescent resin allowed the pore space to be observed microscopically.
Ductile shear-zone: The ductile shear-zone at VT 420 is 0.15 – 0.90 m thick, strikes WSWENE and dips steeply to the SSE and trends parallel to the cleavage (S-zone). The shear direction is sub-vertical, parallel to the mineral stretching lineation. Looking to the E, the shear sense is anti-clockwise, meaning the shear-zones have accommodated thrust displacements. A minimum value for the shear displacement across the shear-zone is 3 m. The structure of the ductile shear-zone can be linked with the Alpine greenschist metamorphism and the development of the regional cleavage. This deformation is purely ductile and includes the formation of a set of mylonitic zones within the shear-zone. Mylonitic zones are mica-rich and more fine-grained than the surrounding rock. The shear-zone is markedly asymmetric, with an abrupt northern boundary (very high deformation gradient) and an indistinct southern boundary.
Brittle deformation: During the course of post-metamorphic regional uplift, the ductile shear-zone was reactivated and underwent brittle deformation. This lead to formation of noncohesive fault breccias several mm thick, particularly in strongly mylonitic zones which acted as pre-existing zones of weakness (reduced mechanical competence, high deformation gradients). The fault breccias can be interpreted genetically as fault gouges and consist of angular rock fragments embedded in a mica-rich matrix. This matrix is non-cohesive because virtually no hydrothermal alteration and cementation of the pore space has occurred after the brittle deformation event. Hypotheses for the origin of the fault gouges besides differential uplift of individual blocks include the effects of periodic movements such as tides and changes in water levels in the nearby reservoirs.
Quantitative porosimetry: A number of methods (Hg injection, water gravimetry, impregnation with polymethylmethacrylate PMMA) have been applied to quantitatively determine the interconnected pore space in the different rock types in the shear-zone. Due to the cohesionless structure, the fault gouge porosity cannot be measured but is estimated at 10 – 30 vol.% from thin-sections. Ultramylonitic rock portions (in which the fault gouges are often embedded) have a rather low porosity (as low as 0.5 vol.%) due to their dense, recrystallized structure. Porosity increases with decreasing degree of ductile deformation, and the granodioritic matrix rocks have open porosities > 1 vol.% in many cases. Porosity of weakly deformed granodiorite decreases with increasing distance from the tunnel wall (10 % decrease over the first 20 cm), which is interpreted as a consequence of microcrack formation during tunnel construction.
Extrapolation of observed structures to larger scales: Because of the limited outcrop size in the tunnel, an attempt to extrapolate the observed structures to larger scales was made using information from geological mapping on the surface. Similar shear-zones at the Räterichsboden reservoir can be mapped on the meter – decametre scale. We assume that the shear-zone geometry described can be extrapolated to the 100 m-range.
Implications for water flow and transport: It is brittle deformation which plays the dominant role as far as water flow is concerned today: the central fault gouge is highly porous due to the lack of hydrothermal cementation. The ultramylonitic rock zones bordering the fault gouge are very tight but do contain planar pore spaces along mica streaks (sheet-silicate porosity). These provide a hydraulic connection between the fault gouge and the less deformed granodioritic gneiss outside the shear-zone. The granodioritic matrix rocks have a well developed, interconnected network of grain boundary and transgranular pores. Feldspars also have numerous solution pores, interpreted as etching phenomena of Alpine metamorphic fluids.
Suggestions for revised conceptual models: Revised conceptual models are suggested for the geometry of the pore spaces and for the hydrodynamic and transport modelling. The geometrical model is based mainly on microscopic parameters derived from thin-sections (for example pore width, persistence and orientation). The model consists of 3 domains: 1) the fault gouges are represented by a system of plates with variable orientation and thickness, consisting of an equivalent porous medium, 2) the mylonitic rocks are described of a highly anisotropic fracture network (sheet silicate pores), 3) the granodioritic gneisses surrounding the shear-zone are represented by a mildly anisotropic fracture network (grain boundary pores). Within these 3 domains, the pore spaces are interconnected. This small-scale conceptual geometrical model can be extrapolated to larger scales by the use of standard averaging techniques. The relevance of advective, dispersive and diffusive transport in all domains was considered. It is suggested that advection and mechanical dispersion is most effective in the fault gouges whereas molecular diffusion is expected to be relevant in the domains 2 and 3. The migration shear-zone can therefore be described in terms of a dual-porosity transport model.